LCC
– 1992: NO named ‘Molecule of the Year’ by the journal Science
– 1997: Creation of the journal Nitric Oxide (impact factor = 4.4)
– 1998: Nobel Prize awarded to NO research
The best NO source agents are ruthenium-nitrosyl complexes (RuNO) which release NO under light irradiation, according to the following reaction:
[RuIIL5NO]n+/- + solvent → [RuIIIL5-(solvent)]n+/- + NO
The intense research activity resulting from their study is illustrated in Figure 1. There has been a fivefold increase in the annual rate of publications over the last 20 years.
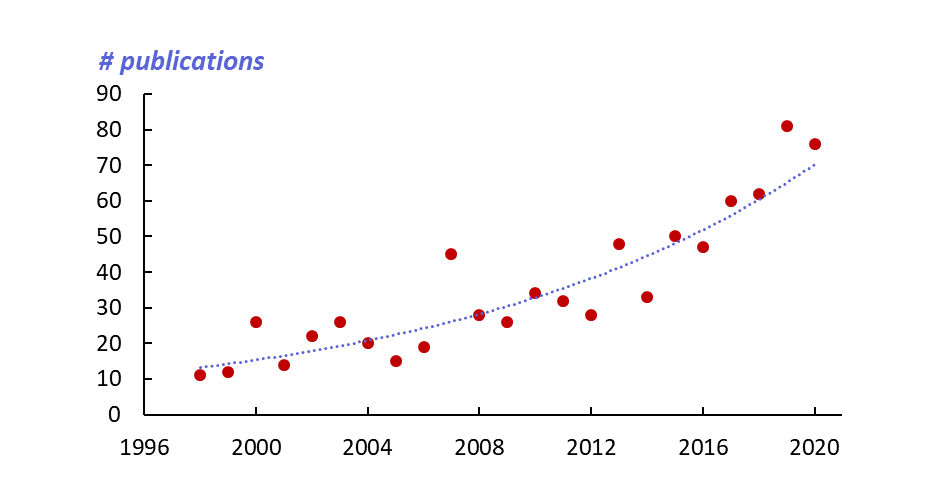
Figure 1: Evolution of the annual rate of publications on RuNO complexes.
(from SciFinder)
Since its creation, the team’s main objective has been the identification of a target molecule for the photo-controlled release of NO for biological applications. The selected system consists of a core [Ru(terpy)(NO)]3+. Some examples are given in Figure 2.
On the basis of these results, various research directions are developed in the team:
- optimization of the molecular property;
- theoretical study of the origin of the properties;
- compatibility of the systems with the biological environment;
- shaping as nano-objects.
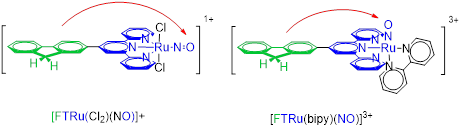
Figure 2:
RuNO complexes showing the Ru(terpy)(NO) core in blue, and the fluorene electron donating in green. Charge transfers from the donor to the NO ligand (highly electron acceptor) shown by red arrows, are responsible for the optical properties and photo-induced release of NO.
1. Optimizing the molecular property
There are two obstacles to the development of the application of RuNO complexes in biological media: (i) the inherent toxicity of NO which requires it to be delivered locally and quantitatively and (ii) the need to use radiation of wavelength = 300 – 500 nm, i.e. outside the relative transparency range of biological tissues ( = 600 – 1300 nm). These pitfalls can be avoided by using two-photon absorption (TPA) in which the transition (300-500 nm) is made by absorbing two photons of double wavelength (600-1000) (see Figure 3). The TPA technique has the added advantage of being localized to the focal point of the radiation, which offers the possibility of working on a targeted cell, thus avoiding collateral damage to healthy tissue.
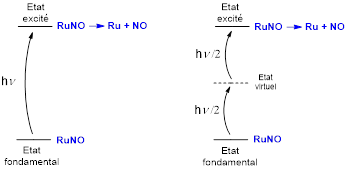
Figure 3: NO release by one-photon absorption (left) or two-photon absorption (right).
An example of the synthesis of a RuNO complex capable of two-photon NO release is shown in Figure 4.
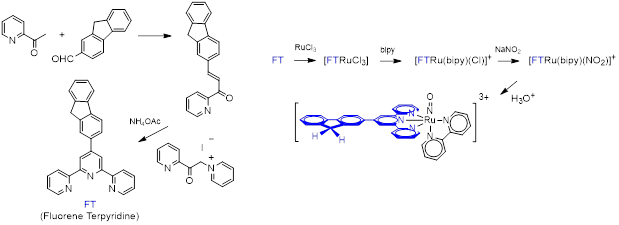
Figure 4: Synthesis of the [FTRu(bipy)(NO)]3+ complex
The TPA properties of these systems are studied by Z-scan, a technique that gives access to the “molecular cross section” (σ) which quantifies the ability of the molecule to absorb two photons in a manner similar to the molar extinction coefficient used for one-photon absorption. σ is expressed in Goppert-Mayer (GM). In the case of monometallic RuNOs the values of vary between 100 and 200 GM. An even more ambitious approach is to study polynuclear RuNOs like those shown in Figure 5.
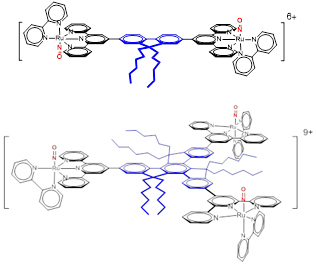
Figure 5: Bimetallic species (up) in C2 symmetry and trimetallic species (down) in C3 symmetry showing enhanced cross sections up to a factor of 16 vs. the reference monometallic species [FTRu(bipy)(NO)]3+. The electron donor fragments are shown in blue and the electron acceptors in red.
2. Theoretical studies
RuNO complexes in which the Ru – N – O conformation is linear present the following electronic structure:
[RuII (NO)+]
If the NO ligand is considered to be charged « + » in its fundamental level, it is invariably observed that the species released corresponds to the NO• radical. In other words, photo-release is accompanied by an electron transfer from the complex to the NO ligand, with the creation of a gap on the ruthenium that is formally oxidized from RuII to RuIII, according to the following reaction:
[RuII (NO)+] → [RuIII•] + NO•
This global scheme appears to be compatible with the study of electronic spectra which reveal the presence of a very intense charge transfer during low energy transitions, with a strong HOMO ® LUMO character (Figure 6).
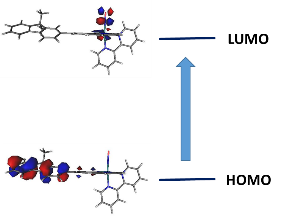
Figure 6 : Frontier orbitals involved in the intense low energy transition of the [FTRu(bipy)(NO)]3+ complex.
This simplified description is however insufficient and several experimental results lead us to carry out a more precise theoretical study on the origin and quantum yield* of photo-release which can vary from one species to another. The studies are conducted using the DFT method (Density Functional Theory) in collaboration with a theoretical chemistry team in Toulouse.
* photo-release quantum yield (φNO) is ratio of the number of NO molecules released to the number of RuNO complexes promoted to the excited level.
3. Compatibility with biological media
The first research on RuNOs carried out in the laboratory aimed to consider these systems only as switchable optical materials. They were then studied in a purely organic medium (acetonitrile) or in the solid state. Looking at them as biological molecules requires studying them in water
The [FTRu(bipy)(NO)]3+ complex is only stable in acidic conditions and transforms to [FTRu(bipy)(NO2)]+ after few minutes at pH=7. However, complexes based on the [Ru(terpy)(Cl)2(NO)]+ stabilize as [Ru(terpy)(OH)(Cl)(NO)]+ (highly stable over a long period in the dark). It is interesting to note that the final compound is the same regardless of the starting isomer, thus avoiding the need for a separation method that can be very difficult to implement. The reaction is illustrated in Figure 7. It is observed for all tridentate substituted terpyridine ligands.
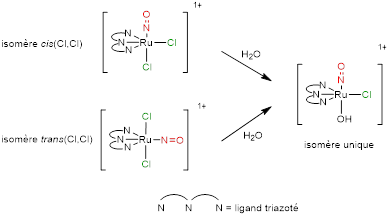
Figure 7: trans(NO,OH) isomer stable in aqueous medium
4. Nano-objects
The use of RuNO complexes in biological tissues poses the delicate question of their shaping (molecules dispersed in solution, grafted onto nanoparticles, dendrimers or polymers, inserted into micellar structures, etc.). Obtaining these objects offers several advantages because, unlike isolated molecules in solution which can only penetrate cells by diffusion, these objects can be captured by the cells by much more efficient mechanisms (e.g. phagocytosis) which lead to a double effect:
– increased penetration of NO carriers
– release of multiple NO units present in the carrier nanocrystal
We develop a nanoprecipitation technique. Aggregates at the nanometer scale of the trans(Cl,Cl)-[RuFTCl2NO]PF6 have been stabilized in water (Figure 8). The photo-release quantum yield is of about 0.12.
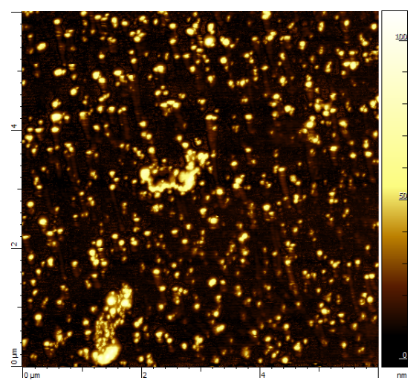
Figure 8: AFM image of nanoaggregates of trans(Cl,Cl)-[RuFTCl2NO]PF6 dispersed in water (30 à 70 nm in size)
LCC
Laboratoire de chimie de coordination du CNRS
205 route de Narbonne, BP 44099
31077 Toulouse cedex 4
France